Prescribed fire is used as a management tool to promote native, fire-tolerant species and reduce vulnerability to high-intensity, unplanned fires. This page discusses how prescribed fire affects ecosystem structure and function, with a primary focus on ecosystem carbon storage and sequestration. Management considerations related to the effects of prescribed fire on ecosystem carbon and greenhouse gas mitigation goals are discussed.
Fire Management: Then and Now
Prior to European settlement, fire was a common disturbance across most of the Midwest and Eastern United States (Pyne 1982, Abrams 1992, Brose et al. 2014, Stambaugh et al. 2015). The frequency and intensity of fire disturbance varied across the region in accordance with climate, topography, vegetation, and management by indigenous people (Thomas-Van Gundy et al. 2020, Abrams and Nowacki 2021). In southern parts of the region, fire was widespread and promoted oak-dominated ecosystems. To the north of the ‘Tension Zone’, more localized burns (particularly on dry sandy soils and near indigenous populations) supported relatively more pine within the extensive mesic conifer-northern hardwood ecosystems (Fig, 1, Nowacki and Thomas-Van Gundy 2024). In the past century or more, widespread fire suppression has shifted the remaining forested ecosystems toward fire-sensitive, shade-tolerant vegetation and reduced landscape heterogeneity. Today, the Midwest and Northeast region is dominated by closed canopy hardwood forests and boasts greater tree density but smaller tree diameters compared to pre-European settlement (Nowacki and Abrams 2008).

Today, prescribed fire is used as a management tool in combination with mechanical thinning to promote native, fire-tolerant species and reduce competition from native, fire-intolerant and non-native, invasive species. Prescribed fire and mechanical thinning are also conducted to reduce vulnerability to high-intensity, unplanned fires. Wildfires burn almost twice as much acreage compared to prescribed fire across the United States, although this pattern varies regionally (National Interagency Fire Center, n.d.). While it is challenging to precisely assess historical fire, it is widely accepted that the extent and frequency of fire in the region prior to European settlement was greater than it is today (e.g., Cleland et al. 2004, Nowacki and Abrams 2008, Eisenberg et al. 2024). Here, we discuss how prescribed fire affects ecosystem structure and function, with a primary focus on ecosystem carbon storage and sequestration. We highlight how effects may differ between prescribed fires and wildfires, as well as between different ecosystems.
Effects of Fire
Fire is a key disturbance in many ecosystems given its effects on vegetation composition, structure, and successional dynamics. Fire directly affects vegetation by killing fire-intolerant species. In the absence of fire, shade- and moisture demanding plant species outcompete fire-tolerant taxa and reinforce site conditions that inhibit fire (e.g., cooler, wetter microclimates; less flammable fuel beds). Fire maintains or increases canopy openness in woody ecosystems and encourages diverse ground flora comprised of light-demanding forbs and grasses. Introducing prescribed fire to a site generally increases ground cover and plant species richness, particularly of native forbs and grasses (Phillips et al. 2007, Cook et al. 2008). Prescribed fire effects on vegetation are often stronger when combined with mechanical thinning (e.g., Lettow et al. 2014, Bowles et al. 2017, Bassett et al. 2020).
Fire alters nutrient cycling directly as well as indirectly via changes in plant productivity and community composition. Fire can release nutrients from soils into the atmosphere, concentrate and deposit nutrients in ash, or leave nutrients as part of incompletely burned biomass (which are then available for plant uptake following decomposition). Fire affects the availability of individual nutrients differently. Nitrogen gasses are emitted at low temperatures while volatilization of phosphorus and cations, such as potassium and calcium, require very high temperatures which are rarely achieved in prescribed fire. As a result, high-intensity fires (typical of many wildfires) result in greater nutrient losses overall and proportionally greater losses of phosphorus and cations compared to prescribed and low intensity fires (Boerner 1982, Zhou et al. 2022). Prescribed or low-intensity fires also combust a smaller proportion of aboveground biomass and convert less nutrients to ash compared to high-intensity fires. Immediately post-fire, there is usually a spike in nitrogen availability that gradually declines due to plant uptake and erosion or leaching (Schlesinger and Bernhardt 2020). Repeated fire generally decreases soil nutrients due to reduced organic inputs and slowed decomposition (e.g., Ojima et al. 1994, Pellegrini et al. 2020), although this is not a universal rule. The plant community response to fire-induced changes in nutrient cycling depends on site fertility and plant composition. For example, long-term reduced nitrogen availability may increase the competitive advantage of native compared to nonnative plant species in systems adapted to low fertility soils. In other sites, reduced nitrogen availability can decrease diversity and productivity.
Fire burns organic matter and releases carbon dioxide into the atmosphere, along with other greenhouse gasses such as methane and nitrous oxide. As such, fire reduces ecosystem carbon storage and increases carbon flux to the atmosphere immediately post-burn. However, the effect of fire on total ecosystem carbon storage (pool size) and sequestration (rate of capturing and securing carbon in the ecosystem) over years to decades is more nuanced (Fig. 2). Assessing fire effects on ecosystem carbon requires consideration of the:
- direct short-term effects of combustion on different carbon pools
- time required to recover short-term carbon losses to pre-fire levels
- fire-induced changes in the a) carbon sequestration potential, b) turnover time (i.e., persistence) of carbon pools, and c) carbon vulnerability to future disturbances
- relative contribution of each carbon pool to total ecosystem carbon storage
Carbon Pools
Each of these points are detailed below. It bears emphasizing that in many cases prescribed fire has minimal effects on longer-term ecosystem carbon cycling.
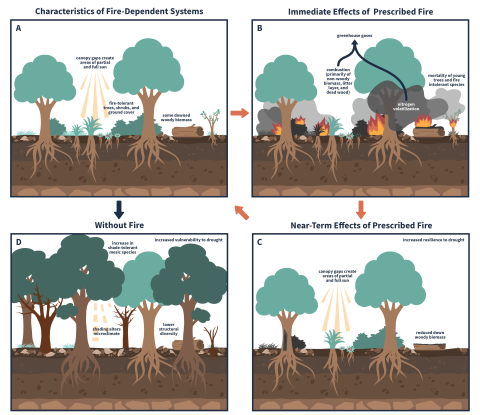
Immediately post-fire, total carbon storage decreases. Carbon stored in live and dead aboveground and litter layer pools decreases more in the short term compared to live belowground and soil carbon pools. Prescribed and low intensity fires primarily affect understory and ground layer vegetation which recover quickly post-fire (months to a few years). By contrast, high-intensity, stand-replacing fires have greater and longer-lasting effects on aboveground carbon storage in woody ecosystems as these pools take decades to recover. Without fire, this biomass would decompose, returning much of the carbon to the atmosphere over decades. For example, brush pile burning emits carbon to the atmosphere in one pulse. If the brush were left to decompose, roughly the same amount of carbon would be emitted to the atmosphere, but as a slow trickle over many years.
The effect of fire on ecosystem carbon storage is dependent on the relative size of each carbon pool (Fig. 3). Because fire has a greater impact on aboveground (compared to belowground) carbon pools, temporary decreases in ecosystem carbon storage due to fire will be greater in systems that store a greater proportion of their carbon in aboveground woody tissues. For example, fire affects grassland carbon storage less than that of forests because grasslands store very little carbon aboveground and don’t have long-lived woody tissues. In forests, combustion of the understory will not dramatically affect forest carbon storage due to the small percentage of total ecosystem carbon this pool represents. Fires reduce forest floor carbon storage in some cases by more than 50%, with bigger decreases from wildfires compared to prescribed fires (Nave et al. 2011). However, forest mineral soil carbon stocks tend to be resistant to fire, suggesting that ecosystem carbon storage will be less impacted by fire in sites with deep mineral soils compared to shallow soil sites with thick forest floors or organic horizons. As such, carbon storage losses due to fire can be quite high in peatlands.


Fire can also affect carbon sequestration potential, persistence, and vulnerability. Collectively these outcomes interact to determine longer-term carbon cycle consequences. In humid climates, vegetation regrowth is often rapid and can result in carbon sequestration rates that match or exceed pre-fire levels in just a few years (e.g., Chiang et al. 2008). Through the process of pyrolysis, carbon molecules are altered and become more resistant to decomposition. Organic matter that, without fire, may have decomposed and emitted carbon dioxide to the atmosphere within decades can persist for centuries or more as pyrogenic carbon, or charcoal, in the soil post-fire (DeLuca and Aplet 2008, Wang et al. 2016). Fire also affects an ecosystem’s carbon sequestration and storage potential indirectly by altering vegetation structure. Frequent fire in fire-adapted ecosystems can prevent woody encroachment. While woody biomass sequesters and holds more aboveground carbon than non-woody vegetation, such fires enhance landscape diversity which can reduce vulnerability to future disturbances and increase climate resilience (Frelich et al. 2020). Prescribed fire can also be employed to reduce the vulnerability of remaining ecosystem carbon to a standreplacing fire. For example, managers can design an initial low-intensity prescribed fire with tight parameters in a densely stocked stand as a first step toward subsequent fires with more variable intensity. Consequently, although prescribed fire may not maximize short-term carbon storage, it can support carbon benefits alongside other management goals while increasing ecosystem resiliency to drought. The suitability of prescribed fire for a given situation will depend upon the historic fire regime, current vegetation community, and management priorities.
Prescribed Fire and Greenhouse Gas Mitigation Goals
Because fire emits carbon into the atmosphere through combustion, a common concern is that prescribed fire contributes to elevated atmospheric carbon dioxide levels and climate change. Scientific research counters this, showing that prescribed fire can support both greenhouse gas mitigation and climate adaptation goals. As described above, prescribed fire typically results in small ecosystem carbon losses that can be recovered relatively quickly and prescribed fire can increase stability of existing carbon pools (e.g., soil carbon) in some ecosystems. Therefore, the net effect of prescribed fire on atmospheric carbon dioxide is generally small to negligible. Additionally, research shows that using prescribed fire on the landscape can reduce the extent and intensity of subsequent wildfires (Hunter and Robles 2020). Large, high-intensity fires emit more greenhouse gases compared to small prescribed fires (Wiedinmyer and Hurteau 2010). Across a landscape, prescribed fire can reduce total ecosystem carbon losses due to fire disturbance while also increasing habitat patchiness and biodiversity.
Considerations for Evaluating the Effects of Management on Ecosystem Carbon
Several main points emerge from the discussion above that can help natural resource professionals consider how prescribed fire will affect ecosystem carbon on a site:
- The current extent and frequency of prescribed fire generally remains below that of historical fire regimes in the region.
- Carbon naturally cycles through an ecosystem. Fire accelerates how quickly carbon in plant biomass returns to the atmosphere.
- The effects of fire on total ecosystem carbon are related to the relative size of the carbon pools most affected, as well as how quickly those carbon pools naturally cycle.
- Fire is most detrimental to greenhouse gas mitigation goals in situations where fire significantly decreases carbon pools that store a lot of carbon for long periods of time and don’t quickly accrue carbon.
- The reintroduction of low-intensity fire can be an important tool for supporting effective climate change adaptation in many landscapes. Its application can be used to both reduce climate change impacts and to restore and enhance ecosystem services that are promoted by fire.
Abrams, M. D.; Nowacki, G. J. 2021. Examining the heritage and legacy of Indigenous land management in oak and pine forests of northeastern United States. International Journal of Ecology and Environmental Sciences, 47: 27–36.
Bassett, T.J.; Landis, D.A.; Brudvig, L.A. 2020. Effects of experimental prescribed fire and tree thinning on oak savanna understory plant communities and ecosystem structure. Forest Ecology and Management, 464: 118047. doi.org/10.1016/j.foreco.2020.118047.
Boerner, R.E.J. 1982. Fire and nutrient cycling in temperate ecosystems. BioScience, 32: 187–192. https://doi. org/10.2307/1308941
Bowles, M.L.; Lawrence, B.A.; Antlitz, D.J. 2017. Thinning effects on canopy structure and ground layer diversity in a burned mesic oak savanna. The Journal of the Torrey Botanical Society, 144: 191–205. doi.org/10.3159/TORREYD-16-00015R1
Brose, P. H.; Dey, D.C.; Waldrop, T.A. 2014. The fire-oak literature of eastern North America: synthesis and guidelines. Gen. Tech. Rep. NRS-135. U.S. Department of Agriculture, Forest Service, Northern Research Station, Newtown Square. 98p. doi.org/10.2737/NRS-GTR-135.
Chiang, J.-M.; McEwan, R.W.; Yaussy, D.A.; Brown, K.J. 2008. The effects of prescribed fire and silvicultural thinning on the aboveground carbon stocks and net primary production of overstory trees in an oak-hickory ecosystem in southern Ohio. Forest Ecology and Management, 255: 1584–1594. doi.org/10.1016/j.foreco.2007.11.016.
Cleland, D.T.; Crow, T.R.; Saunders, S.C.; Dickmann, D.I.; Maclean, A.L.; Jordan, J.K.; Watson, R.L.; Sloan, A.M.; Brosofske, K.D. 2004. Characterizing historical and modern fire regimes in Michigan (USA): A landscape ecosystem approach. Landscape Ecology, 19: 311–325. doi.org/10.1023/B:LAND.0000030437.29258.3c.
Cook, J. E.; Jensen, N.; Galbraith, B. 2008. Compositional, cover, and diversity changes after prescribed fire in a mature eastern white pine forest. Botany, 86: 1427–1439. doi.org/10.1139/B08-111
Domke, G.M.; Perry, C.H.; Walters, B.F.; Woodall, C.W.; Russell, M.B.; Smith, J.E. 2016. Estimating litter carbon stocks on forest land in the United States. Science of The Total Environment, 557-558: 469–478. doi.org/10.1016/j. scitotenv.2016.03.090
DeLuca, T. H.; Aplet G.H. 2008. Charcoal and carbon storage in forest soils of the Rocky Mountain West. Frontiers in Ecology and the Environment, 6: 18–24. doi.org/10.1890/070070.
Eisenberg, C.; Prichard, S.; Nelson, M. P.; Hessburg, P. 2024. Braiding Indigenous and Western Knowledge for ClimateAdapted Forests: An Ecocultural State of Science Report. depts.washington.edu/flame/mature_forests/pdfs/ BraidingSweetgrassReport.pdf
Frelich, L. E.; Jõgiste, K.; Stanturf, J.; Jansons, A; Vodde, F. 2020. Are secondary forests ready for climate change? It depends on magnitude of climate change, landscape diversity and ecosystem legacies. Forests, 11: 965. doi. org/10.3390/f11090965
Hunter, M.E.; Robles, M.D. 2020. Tamm review: The effects of prescribed fire on wildfire regimes and impacts: A framework for comparison. Forest Ecology and Management, 475: 118435. doi.org/10.1016/j.foreco.2020.118435.
Jackson, R.B.; Canadell, J.; Ehleringer, J.R.; Mooney, H.A.; Sala, O.E.; Schulze, E.D. 1996. A global analysis of root distributions for terrestrial biomes. Oecologia, 108: 389–411. doi.org/10.1007/BF00333714.
Lettow, M.C.; Brudvig, L.A.; Bahlai, C.A.; Landis, D.A. 2014. Oak savanna management strategies and their differential effects on vegetative structure, understory light, and flowering forbs. Forest Ecology and Management, 329: 89–98. doi.org/10.1016/j.foreco.2014.06.019.
Liu, S.; Liu, J.; Young, C.J.; Werner, J.M.; Wu, Y.; Li, Z.; Dahal, D.; Oeding, J.; Schmidt, G.L.; Sohl, T.L.; Hawbacker, T.J.; Sleeter, B.M. 2012. Baseline carbon storage, carbon sequestration, and greenhouse-gas fluxes in terrestrial ecosystems of the western United States. In: Zhu, Z.; Reed, B.C., eds. Baseline and projected future carbon storage and greenhouse-gas fluxes in ecosystems of the western United States. U.S. Geological Survey Professional Paper 1797. Reston, VA: U.S. Department of Interior, Geological Survey. 192p.
National Interagency Fire Center [NIFC]. nifc.gov. (accessed April 8, 2024).
Nave, L.E.; Vance, E.D.; Swanston, C.W.; Curtis, P.S. 2011. Fire effects on temperate forest soil C and N storage. Ecological Applications, 21: 1189–1201. doi.org/10.1890/10-0660.1.
Nowacki, G.J.; Abrams, M.D. 2008. The demise of fire and “mesophication” of forests in the eastern United States. BioScience, 58(2): 123–138. doi.org/10.1641/B580207.
Nowacki, G. J.; Thomas-Van Gundy, M.A. 2024. Using witness trees as pyro-indicators to depict past fire environments across the eastern United States. Fire Ecology, 20: 13. doi.org/10.1186/s42408-024-00247-1.
Ojima, D.S.; Schimel, D.S.; Parton, W.J.; Owensby, C.E. 1994. Long- and short-term effects of fire on nitrogen cycling in tallgrass prairie. Biogeochemistry, 24: 67–84. doi.org/10.1007/BF02390180.
Pellegrini, A.F.A.; Hobbie, S.E.; Reich, P.B.; Jumpponen, A.; Brookshire, E.N.J.; Caprio, A.C.; Coetsee, C.; Jackson, R.B. 2020. Repeated fire shifts carbon and nitrogen cycling by changing plant inputs and soil decomposition across ecosystems. Ecological Monographs, 90: e01409. doi.org/10.1002/ecm.1409.
Phillips, R.; Hutchinson, T.; Brudnak, L.; Waldrop, T. 2007. Fire and fire surrogate treatments in mixed-oak forests: effects on herbaceous layer vegetation. In: Butler, Bret W.; Cook, Wayne, comps. The fire environment-- innovations, management, and policy; conference proceedings. 26-30 March 2007; Destin, FL. Proceedings RMRS-P46CD. Fort Collins, CO: U.S. Department of Agriculture, Forest Service, Rocky Mountain Research Station. p. 475–485.
Pyne, S.J. 1982. Fire in America: a cultural history of wildland and rural fire. University of Washington Press. 680 p. jstor.org/stable/j.ctvcwnkj3.
Schlesinger, W. H.; Bernhardt, E. 2020. Biogeochemistry: an analysis of global change. 4th edition. London: Academic Press. 762 p.
Stambaugh, M.C.; Varner, J.M.; Noss, R.F.; Dey, D.C.; Christensen, N.L.; Baldwin, R.F.; Guyette, R.P.; Hanberry, B.B.; Harper, C.A.; Lindblom, S.G.; Waldrop, T.A. 2015. Clarifying the role of fire in the deciduous forests of eastern North America: reply to Matlack: fire in deciduous forests. Conservation Biology, 29: 942–946. doi.org/10.1111/ cobi.12473.
Thomas-Van Gundy, M. A.; Nowacki, G. J.; Anderson, R. C.; Bowles, M. L.; Brugam, R. B.; Pavlovic, N. B.; Halsey, S. J.; Mcbride, J. 2020. Visualizing the ecological importance of pre-Euro-American settlement fire across three midwestern landscapes. The American Midland Naturalist, 183: 1–23. doi.org/10.1637/19-030.
Wang, J.; Xiong, Z.; Kuzyakov, Y. 2016. Biochar stability in soil: meta-analysis of decomposition and priming effects. Global Change Biology Bioenergy, 8: 512–523. doi.org/10.1111/gcbb.12266.
Wiedinmyer, C.; Hurteau, M.D. 2010. Prescribed fire as a means of reducing forest carbon emissions in the western United States. Environmental Science & Technology, 44: 1926–1932. doi.org/10.1021/es902455e.
Woodall, C.W.; Walters, B.F.; Oswalt, S.N.; Domke, G.M.; Toney, C.; Gray, A.N. 2013. Biomass of carbon attributes of downed woody materials in forests of the United States. Forest Ecology and Management, 305: 48–59. doi. org/10.1016/j.foreco.2013.05.030.
Zhou, Y.; Biro, A.; Wong, M.Y.; Batterman, S.A.; Staver, A.C. 2022. Fire decreases soil enzyme activities and reorganizes microbially mediated nutrient cycles: A meta-analysis. Ecology, 103: e3807. doi.org/10.1002/ ecy.3807.
Suggested Citation of the Hub Publication
Keller, A.B. and Handler, S. 2024. Effects of fire on ecosystem carbon in the Midwest and Eastern United States. Technology Transfer. Houghton, MI: U.S. Department of Agriculture, Northern Forests Climate Hub. 8 p. https://doi.org/10.32747/2024.8633530.ch
Acknowledgments
This is a product of the USDA Northern Forests Climate Hub and the Northern Institute of Applied Climate Science, a collaborative, multi-institutional partnership led by the USDA Forest Service. Funding was provided by the USDA Forest Service and The Nature Conservancy.